types of insect wings pdf
Summary
Dive into the fascinating world of insect wings! Download our PDF guide and explore the diverse types, structures, and evolution of these amazing flyers. Get your insect wings knowledge now!
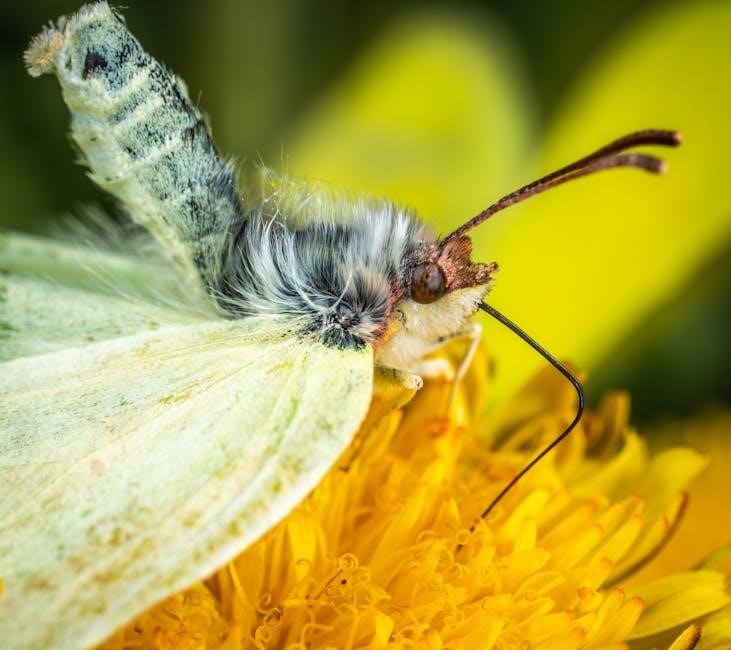
Insect wings are vital for survival, adapting to diverse ecological roles․ Their varied forms, like elytra and halteres, reflect adaptations to different flight styles and environments․ Understanding wing types reveals evolutionary insights into insect flight and ecology․
Overview of Insect Wing Morphology
Insect wing morphology is a complex field, examining the size, shape, and structure of wings, which are intrinsically linked to flight capabilities․ Systematic studies reveal variability in natural patterns across insect species, employing topological and geometric analyses․ Key aspects include wing size, contour shape, and vein geometry, providing insights into function and evolution․
Wings, as adult outgrowths of the exoskeleton, are typically found on the mesothorax and metathorax, often referred to as forewings and hindwings․ Strengthened by longitudinal veins, these structures showcase diverse modifications related to specific lifestyles and environmental demands․ Variations in morphology provide essential clues about evolutionary adaptations and ecological roles․
Furthermore, understanding wing morphology involves considering the homology of wing areas and the functions of longitudinal bending lines․ Distinctions between flexion-lines and fold-lines elucidate aerodynamic function and wing-folding mechanisms․ Insect wing venation patterns, wing contour, and vein-bounded domain shapes are also crucial elements․
Basic Wing Structures
Insect wings feature longitudinal veins providing support, connected by cross-veins․ These veins form a framework, essential for wing strength and flexibility during flight․ Understanding these structures is crucial to deciphering wing function․
Longitudinal Veins
Longitudinal veins are the primary supporting structures within an insect wing, extending from the base to the distal margin․ These veins are not merely structural; they also play a critical role in the hemolymph circulation, facilitating nutrient transport throughout the wing․ Typically, six main longitudinal veins are identified, each with its unique branching pattern and contribution to wing stability․
The naming convention for these veins, from anterior to posterior, includes Costa (C), Subcosta (Sc), Radius (R), Media (M), Cubitus (Cu), and Anal veins (A)․ These veins are not always present in all insects, and their arrangement can vary significantly depending on the species and evolutionary adaptations․ The Costa vein, located along the leading edge of the wing, provides rigidity and resistance to aerodynamic forces․
The Radius and Media veins are often branched, forming a complex network that supports the wing membrane and enhances its aerodynamic properties․ The Cubitus and Anal veins are typically less branched but contribute to the overall structural integrity of the wing․ These longitudinal veins are essential for flight, providing the necessary framework and flexibility for insects to maneuver effectively․
Cross-Veins
Cross-veins are secondary veins in insect wings that connect the longitudinal veins, forming a network-like structure that enhances wing strength and rigidity․ Unlike longitudinal veins, cross-veins do not extend from the base to the tip of the wing; instead, they bridge the spaces between the major longitudinal veins․ These veins play a critical role in preventing wing deformation during flight by distributing stress across the wing surface․
The arrangement and number of cross-veins can vary significantly among different insect species, contributing to the diversity of wing venation patterns․ These variations often reflect adaptations to specific flight behaviors and ecological niches․ Cross-veins are named based on the longitudinal veins they connect, such as the radio-medial cross-vein (r-m) connecting the Radius and Media veins, and the medio-cubital cross-vein (m-cu) connecting the Media and Cubitus veins․
The presence and arrangement of cross-veins influence the flexibility and aerodynamic properties of the wing, allowing insects to fine-tune their flight performance․ This intricate network of veins ensures that the wing can withstand the aerodynamic forces generated during flight, providing stability and control․
Wing Modifications and Adaptations
Insect wings exhibit diverse modifications, driven by ecological demands․ Adaptations like elytra and halteres showcase nature’s ingenuity․ These changes enhance survival through specialized functions, reflecting the remarkable evolutionary history of insects․
Forewing Modifications
Forewings in insects often undergo significant modifications, reflecting diverse adaptations to ecological niches․ Elytra, found in beetles (Coleoptera), are hardened forewings that serve as protective covers for the delicate hindwings and abdomen․ These rigid structures offer substantial defense against predators and environmental damage, allowing beetles to inhabit harsh environments․
Hemelytra, characteristic of true bugs (Hemiptera), are forewings with a basal portion that is thickened and hardened, while the apical portion remains membranous․ This unique design provides both protection and aerodynamic functionality․ Tegmina, seen in grasshoppers and related insects, are leathery forewings that offer camouflage and some protection during flight․
In some moths and butterflies, the forewings are covered in scales, contributing to their vibrant colors and patterns, which aid in mate recognition and predator avoidance․ These modifications demonstrate the remarkable adaptability of insect forewings in response to selective pressures, enhancing their survival and reproductive success․ The diverse forms reflect a complex interplay between structure and function, showcasing evolutionary innovation;
Hindwing Modifications
Hindwings in insects exhibit a range of modifications that complement forewing function and adapt to specific flight requirements․ Halteres, found in flies (Diptera), are reduced hindwings that act as gyroscopic balancing organs․ These small, club-shaped structures oscillate during flight, providing sensory feedback that helps stabilize the insect and maintain equilibrium․ This adaptation allows for highly maneuverable and precise flight, crucial for navigating complex environments․
In some insects, hindwings are larger and more membranous than forewings, providing the primary propulsive force for flight․ These wings often have intricate folding patterns that allow them to be neatly tucked away when not in use․ Fringed hindwings, common in small insects like thrips, have long, hair-like structures along the margins, increasing surface area and enhancing aerodynamic efficiency․
The modifications of hindwings demonstrate the evolutionary pressures that shape insect flight, optimizing performance for diverse ecological niches․ From balance to propulsion, these adaptations highlight the intricate relationship between wing structure and functional demands, contributing to the success of insects in the air․
Types of Insect Wings
Insect wings exhibit remarkable diversity, adapted for flight, protection, or sensory functions․ Key types include membranous wings for efficient flight, elytra for protection, and halteres for balance, showcasing evolutionary adaptations to different ecological niches․
Membranous Wings
Membranous wings are the most common type found in insects, characterized by their thin, transparent structure․ These wings are primarily used for flight and are supported by a network of veins that provide structural integrity․ The veins also play a role in nutrient transport and sensory functions․ Insects with membranous wings typically have two pairs, although some species may have reduced or absent hindwings․
These wings are highly efficient for flight, allowing for maneuverability and sustained aerial movement․ The wing surface is covered with tiny hairs or scales in some species, which can influence aerodynamic properties․ Membranous wings are found in a wide range of insect orders, including dragonflies, damselflies, bees, wasps, and flies․ The venation patterns in membranous wings are often used for taxonomic identification․
The evolution of membranous wings represents a significant adaptation for insects, enabling them to exploit new ecological niches and disperse over long distances․ Their lightweight and flexible structure allows for efficient flight with minimal energy expenditure․ Modifications in wing shape and size can further enhance flight performance, adapting insects to specific environments and lifestyles․
Halteres
Halteres are modified hindwings found in insects of the order Diptera (true flies)․ These small, club-shaped structures act as gyroscopic balance organs, providing sensory feedback to the insect during flight․ Halteres oscillate in a plane opposite to the forewings, detecting changes in body orientation and helping the fly maintain stability․
The halteres are equipped with sensory receptors called campaniform sensilla, which detect minute changes in the haltere’s position․ This information is relayed to the flight muscles, allowing the fly to make rapid adjustments and maintain a stable flight path․ The presence of halteres is a defining characteristic of Diptera, distinguishing them from other winged insects․
The evolution of halteres represents a significant adaptation for flies, enabling them to perform complex aerial maneuvers and hover with precision․ These structures are crucial for maintaining balance and coordination during flight, particularly in windy or unstable conditions․ The study of halteres has provided valuable insights into the biomechanics of insect flight and the evolution of sensory systems․
Elytra
Elytra are hardened forewings found in beetles (order Coleoptera)․ These modified wings serve as protective covers for the delicate hindwings and abdomen․ Elytra are typically thick and opaque, providing a shield against physical damage and environmental stressors․
During flight, elytra are held aloft, allowing the membranous hindwings to unfold and propel the beetle through the air․ The rigid structure of elytra offers additional support and protection during takeoff and landing․ The surface of elytra can vary greatly, exhibiting diverse textures, patterns, and colors, often serving as camouflage or for species recognition․
The evolution of elytra represents a key adaptation for beetles, enabling them to exploit a wide range of terrestrial habitats․ Their protective function allows beetles to thrive in environments where other insects might be vulnerable․ The study of elytra provides insights into the evolutionary pressures that have shaped the diversity of beetles and their ecological roles․
Hemelytra
Hemelytra are characteristic forewings found in true bugs (order Hemiptera)․ These wings are partly hardened and partly membranous, hence the name “hemi” (half) and “elytra” (hardened wing)․ The basal portion of the hemelytron is thickened and leathery, providing protection, while the distal portion is membranous, allowing for flight․
When at rest, the hemelytra are held flat over the abdomen, with the membranous tips overlapping․ This unique wing structure provides both protection and flight capability, enabling hemipterans to exploit various ecological niches․ The hardened portion of the hemelytron shields the delicate hindwings and abdomen from damage․
Hemelytra are a key diagnostic feature of Hemiptera, distinguishing them from other insect orders․ The venation patterns on the membranous portion of the hemelytron can be used for species identification․ The evolution of hemelytra represents an adaptation to diverse feeding strategies and habitats, contributing to the ecological success of true bugs․
Fringed Wings
Fringed wings, also known as plumose wings, are a unique type of insect wing characterized by long, hair-like setae along the margins․ These fringes significantly increase the wing surface area, enhancing flight efficiency in small insects․ Thrips (order Thysanoptera) are the primary group exhibiting this wing type․
The fringed wings of thrips allow them to remain airborne despite their small size, enabling them to disperse and colonize new habitats effectively․ The setae create a paddle-like effect, increasing drag and lift, which is essential for stable flight․ These wings are typically narrow and lack prominent venation․
The evolution of fringed wings in thrips represents an adaptation to their minute size and specialized feeding habits․ These insects often feed on plant sap or pollen, and their fringed wings facilitate access to confined spaces․ Fringed wings are an excellent example of how wing morphology can be modified to suit specific ecological niches․
Wing Venation Patterns
Wing venation patterns are the arrangement of veins in insect wings․ These patterns offer structural support and are crucial for insect identification and evolutionary studies․ Variations in venation reflect adaptations to different flight requirements;
Archedictyon and Evolutionary Changes
The Archedictyon represents a hypothetical ancestral wing venation pattern, suggesting all winged insects evolved from a common ancestor․ This concept is fundamental to understanding the evolutionary modifications of insect wings over millions of years․ The Archedictyon serves as a template, demonstrating how natural selection has streamlined and adapted wing structures․
Evolutionary changes to the Archedictyon have resulted in diverse venation patterns observed in modern insects․ These modifications reflect adaptations to various ecological niches and flight requirements․ Selective pressures have driven the simplification, reduction, or elaboration of veins․ Intermediate forms demonstrate transitional stages in wing morphology․
Secondary wing reductions among pterygotes indicate relaxed selective pressures․ Studying these transitions provides insights into the evolutionary trajectories of wing development․ The venation patterns observed in insect wings reflect the influence of environmental factors and the necessity for efficient flight․ Understanding these patterns is critical for systematic studies and morphological analyses, aiding in the classification and evolutionary understanding of insects․ Furthermore, the Archedictyon provides a foundation for exploring wing function and evolution in relation to insect behavior and ecology․
Wing Function and Flight
The size, shape, and structure of insect wings are intimately linked to their ability to fly, with wing morphology playing a crucial role in aerodynamic performance․ Insect flight involves complex movements, where wing deformation enhances flight stability and efficiency․ Relief in the wing structure supports its shape during flight, with corrugation and specialized sections contributing to aerodynamic support․
Longitudinal lines of bending in the wings also play a crucial role, distinguishing between flexion-lines, which are primarily aerodynamic in function, and fold-lines, which facilitate wing-folding․ The nature, occurrence, and morphological basis of insect wing deformation in flight are critical for understanding flight mechanics․ The evolutionary modifications of insect wings reflect adaptations to enhance flight performance under various environmental conditions․
Blood flow through wing veins contributes to wing functionality, though it primarily transports nutrients and hormones rather than oxygen․ Understanding wing venation patterns is essential for investigating wing function and evolution․ The study of wing morphology provides insights into the biomechanics of flight, with research focusing on topological and geometric analyses to understand wing size, shape, and vein geometry․ These studies contribute to a comprehensive understanding of insect flight dynamics and evolutionary adaptations․